Tesla’s 3-Phase 4-Pole AC Induction Motor — Why Nikola Tesla’s 19th Century Induction Motor Is The Ideal Choice For The 21st Century Electric Car

Sign up for daily news updates from CleanTechnica on email. Or follow us on Google News!
Pop quiz: You’re at a cocktail party. Inevitably, the conversation turns to electric cars. An attractive member of the opposite sex leans over to you, sizes you up as someone who knows their amps from their volts, focuses a pair of bedroom blue eyes in your direction that burn a hole through you like a laser beam, and in a soft, suggestive voice says, “Hey, what’s up with Tesla Motors using a 3-phase 4-pole AC induction motor to power their cars? Why did they go that route?”
Still recovering from the glance, you reply with an Elon-esque stammer, “Uh.. uh.. yes, I.. I can tell you all about that.” As your brain unfreezes, the answer rolls off your tongue like poetry and soon you and your inquisitor are on the dance floor making beautiful music together.
Unless you were an idiot and forgot to prep for such an inquiry before donning your tuxedo. That’s where this helpful “Primer For The 3-Phase 4-Pole AC Induction Motor” comes in. It’s a quick study on how to respond to that very question. Moreover, this handy dandy guide trains you to reply with an answer keyed to the precise geek level of the questioner. You want to refrain from overwhelming your acquaintance with undue mood-killing minutia … but you don’t want to come up short on details if they are requested. That’s just as much of a showstopper.
Let’s start with the basics. The shortest possible answer to the question of why Tesla Motors uses a 3-phase 4-pole AC induction motor to power all its cars is of course “because.” If this works, fine. Move to the dance floor. If a further response is required, a slightly more elaborate because is that the prototype EV that Tesla Motors licensed the technology to was already using an AC motor. Furthermore, it’s been pointed out that if a DC motor had been used, then Tesla Motors couldn’t be called Tesla Motors, given that Nikola Tesla was into AC, not DC.
Geek Level 1
If further explanation is required, you are likely dealing with a geek of some varying magnitude. So let’s kick it up a notch. It’s time to supply this person with the actual advantages of the AC induction motor over your garden-variety DC equivalent. They are:
- Simplified design
- Lower cost
- Fewer parts
- Higher reliability
- Less chance of raw material price fluctuations and shortages
One reason for higher reliability is that a part found on older-style DC motors called a brush has a limited life. A pair of brushes physically engage a rotating shaft and friction occurs. Thus, the brushes wear out long before the motor’s end of life is reached.
The other significant parts eliminated were permanent magnets. The kind of magnets often used in electric motors are so-called rare earth magnets, which are quite powerful. China has the bulk of rare earth deposits and in the past has restricted their export. Besides being expensive, permanent magnets in general add weight to the motor, are subject to demagnetizing and breakage, and there are alternatives that allow for more control over the magnetic fields they produce. It is in fact AC current that actually substitutes for the magnets.
So, the elimination of parts subject to breakage or shortages, the reduction in total parts (and their associates costs), and a lighter-weight motor all present compelling arguments. Perhaps now the conversation can move to the dance floor. If not, bigger guns can be brought to bear.
Geek Level 2
OK. Well, the problem with simply summarizing the advantages of the AC induction motor is that the summaries don’t explain what was used to substitute for the brushes, or what it is about Nikola Tesla’s 136-year-old invention that allowed the magnets to come out.
Before going further, it helps to understand one basic (and very cool) thing about electric motors. AC or DC, they are all about magnetic fields. Magnetism is the name of this game. The Electromagnetic Force is one of the four fundamental forces of nature (the others being the Gravitational Force, the Strong Force [holds atoms together], and the Weak Force [decay of fundamental particles]).
Electromagnetism is the superhero force that makes motors spin. Just think for a second about how two magnets facing each other with the same polarity tend to repel, and likewise how magnets pull together when opposite polarities are facing (opposites attract). So imagine if you were to duct tape a bunch of magnets to, say, a Lazy Susan, and then place a big magnet near that bundle. What would happen? That’s basically how electric motors work. And the bigger the magnets, the more powerful the force that spins the cylinder. Whether powered by magnets or an ICE, the twisting force that spins a motor is termed torque. In the case of electric motors, the stronger the magnetic fields, the stronger the torque.
When designing an electric motor, there are several ways to come up with these all-important magnetic fields. One way is with natural (permanent) magnets. Some minerals are naturally magnetized and emit an electromagnetic field. On the other hand, if you go to the trouble of formulating an alloy using the rare-earth element neodymium, you get a very serious magnet that motor designers love (more torque). A second way to make a magnetic field is to take a few wrappings of copper wire and apply a DC (direct current) voltage to the winding. The coil will emit an electromagnetic field. Thirdly, you can create an electromagnetic field with AC (alternating current), but we’re going to save that one for a moment. Given what’s been said, we now have enough of a primer to talk about brushes, magnets, and AC induction motors in a way that will hopefully make sense and not be some abstract notion.
Brushes
In simple terms, conventional DC motors work by surrounding a rotating shaft with windings of copper wire that are energized by a DC power source (termed an armature because the wires are wrapped around the arms of a metal frame). The rotor is then surrounded by another magnetic field from a fixed position, which is accomplished with permanent magnets attached to a metal case encircling the rotor (this sort of rig is called a stator because it is stationary).
The problem is how to get power to the coil on the rotor since it will be spinning (to make the wheels on the car go round and round). This is where brushes come in. Pressing a conductive material against the rotor does the job, as illustrated here:
As you can see, a pair of carbon brushes are connected to the DC power leads. The brushes make physical contact with a designated area near the end of the rotor that distributes the voltage to the armature (it’s called a commutator because it commutes the current across). The weakness of this design is that you have two parts rubbing against each other and the [softer] brushes eventually wear down to a nub and must be replaced. You don’t want that happening in traffic, which is why a brushed motor is a non-starter in an EV.
Note: From a green perspective, you don’t want to be around a brushed motor. As the brushes wear down, they spew nasty particles of graphite into the air. Brushed motors contribute in some small way to air pollution.
Now, as it happens, the DC motor evolved some time ago into a version not requiring brushes. Some clever person had the brilliant idea of moving the permanent magnets from the stator to the rotor, and moving the coils of wire from the rotor to the stator. With this advance, the DC leads could easily be attached to the coils on the stationary part of the motor. There was no longer a need to get electricity to the rotor. So, out went the brushes and the commutator.
Magnets
However, the magnets still remained. For all the reasons cited above, they need to go too. And this is where we get the opportunity to comprehend the brilliance of the AC induction motor used in a Tesla.
Note: the induction motor was also used in the EV-1. Modern hybrids, however, all use brushless DC motors.
Enter AC or alternating current. Unlike DC voltage, which has constant polarity (like a AA battery with a positive and a negative end), AC changes from positive to negative several times a second (it alternates the polarity from + to -). AC is measured as an oscillating sine wave. To take household wiring as an example, the voltage goes from zero to a positive 120 volts … then ramps down to zero volts. It then repeats the cycle, only with the polarity reversed. This occurs 60 times a second in the US and 50Hz in Europe. Here’s an image of an AC sine wave:
So, why do you care? Because each time the voltage rises and falls, an electromagnetic field is created. It’s the change in voltage levels that creates the field. This is a new, third way, to make a magnetic field. Great. But how does this help our motor? Recall that you want neither coils of wire nor magnets around the rotor. So what you do instead is use a casting of conductive material such as aluminum or copper for the rotor, and you energize opposing pairs of coils in the stator with alternating current instead of direct current. A pair of coils (180° apart) in the stator are wired in series (Christmas tree lights). A wire is wrapped around a steel core in a clockwise manner, which is then extended to the opposing coil and wrapped in a counter-clockwise manner.
When an AC voltage is applied to the pair of coils, an electromagnetic field of opposite charges is created. The fields extend to each other across a plane of the conductive rotor, creating an electromagnet with a north and south pole. This activity induces a current to flow on the rotor. The induced current ebbs and flows along with the electromagnetic field from the AC source. As the rotor current rises and falls, guess what happens? For the same reason current flowing in the coils produced an electromagnetic field, the current flowing on the rotor does the same.
Did you get that? It is the key to how induction motors dispense with brushes and magnets. Without having to understand the physics of what creates an electromagnetic field or how an electromagnetic field could induce a current flow, the point is that, all of a sudden, the rotor is creating a magnetic field in tune to the cycling of the AC current feeding the stator coils. Without all the fuss of brushes and rare earth magnets, we’re back to having the all-important two opposing magnetic fields that make a motor spin.
So, once again: Paired coils on the stator > apply an alternating current to the coils > an electromagnetic field is created that spans the rotor > a current is INDUCED to flow on the rotor > the rotor emits an opposing electromagnetic field > the rotor turns in response to the magnetic pull. Voilà. The AC induction motor. Here’s an animation of that principal. Scroll down to “How does an AC induction motor work?”
Thank you Nikola Tesla for figuring that out!
Tesla uses a copper rotor for its motors in lieu of aluminum due to copper’s much better conductivity. It’s a more difficult and costlier process, though. The copper must be heated to a molten state and then poured into a die with an iron/steel core (known as die casting). Aluminum melts at 660°C whereas Copper melts at 1000°C. The copper must be poured without melting the die or the core. Only recently was this process perfected. The result is an efficient motor which is ~20% smaller than an equivalent aluminum motor.
Geek Level 3
OK. Had enough? On the dance floor yet? Oh, they still want to know about the 3-phase 4-pole part, eh? Alright. Starting with the “4-pole” thing…. The earlier animation demonstrates a 2-pole motor. Just two opposing coils are wired together (in series). Thus, two poles, or a 2-pole motor. The animation has two sets of those paired coils (blue and red), and real motors will have several more around the circumference of the stator, but it is still considered a 2-pole motor.
A refined version of this design uses 4 coils wired in series. One paired set of poles still face opposite each other, but another pair is added 90° apart. Thus, the 4-pole motor. Doubling the number of poles in the circuit increases torque. Picture an apple pie cut in four slices. That gives you an idea of the topography of the coils, which sit at the fat end of each slice. As noted with 2-pole motors, there will be multiple sets of these 4 coils, wired in the same manner. You can just keep slicing that pie up in your head or look at this fully wired stator:
It’s pretty hard to tell how many poles this stator has. It all depends on how the coils were wired together. What you’re looking at is insulated copper wire wrapped around the length of the stator. The coils of wire are referred to as windings for obvious reasons.
Now for the 3 phase thing. 3 phase AC is just three sources of AC power. It takes the form of three separate power cables. Think of AC phases as cylinders in a conventional ICE. The more cylinders (power sources), the smoother the engine runs. Right? A V8 engine runs smoother than a 4 cylinder engine and has more power. Likewise, you get more work done with 3-phase power than the typical single-phase source such as from a household outlet. But how best to apply the extra power? The earlier image of a sinewave was of a single phase circuit. Let’s look at a sinewave again before continuing:
Notice that power starts at zero volts, drops to zero volts as the cycle transitions from positive to negative, and then drops to zero volts again. No voltage = no current flow in that instant. No current flow equals no electromagnetic field. So, with our example 60Hz power, there is no power 120 times each second. Aahh! That will never do for a Tesla. The solution to this “problem” is to apply a separate AC source to power an adjacent set of coils. But you don’t power both circuits at the same time. Instead, you time it so that the second AC source is producing an electromagnetic field when the first is not. Thus, the motor is always powered. And what the heck, if two AC legs are good, three must be better (3 phase is the industry standard). So, now, three separate AC sources are each powering a separate set of 4 coils (4 poles, at 4 opposing points on the compass) at any given moment, each out of phase with the other.
To continue our ICE analogy, do the pistons in the engine all have their combustion cycle occur in the same instant? No. The combustion cycles are staggered (phased) to maximize torque and smooth the operation of the engine. It’s the very same principal for electric motors. The below diagram illustrates 3 phase AC in action. Notice that, at any point in time, voltage is present… thus current flow… thus an electromagnetic field… thus a force pulling on the rotor.
Add it all up and, in effect, you have what designers term a rotating magnetic field progressing around the circumference of the rotor. The rotor is constantly being dragged around in a circle, never quite catching up to the rotating field. The action is orchestrated by an electronic controller.
So, there it is. The 3-phase 4-pole induction motor. A simple device actually. Steel. Copper. Windings. No friction from moving parts except the bearings the rotor turns on. It’s the alternating current that really makes the magic happen…. the movement of those electromagnetic fields creating opposing forces that twist the rotor in circles. It’s all sort of a dance when you think about it. And speaking of dancing, you certainly should have your partner on the floor by now. Don’t make me go to geek level 4!
Thanks to Ivan over at Ivan’s Garage for filling in the blanks of my knowledge. Ivan winds his own motors, so he knows a thing or two about the AC inductive motor.
More References:
Wally Rippel, who helped develop the motor for the EV-1 and later worked at Tesla Motors, talks motors in this blog post.
Another great blog thread is from 2006, where Tesla co-founder Martin Eberhard discusses induction motors.
Here’s a great video from a show called “How It’s Made — Dream Cars” showing a detailed assembly of a Model S. At 5:30 min in, they show the motor being assembled. One note: The narrator states: “This is a 3 phase motor so there are 3 separate coils of copper”. Hmmm. It’s probably more accurate to state that 3 separate coils of copper are energized at the same time.
And here is an article on copper rotors with quotes from JB Straubel.
Or, how about an older animation explaining how magnetism works? Good for kids too.
Here’s a video of a Tesla drive unit on display in the showroom of a Tesla store in Boulder Colorado:
Lastly, here’s a YouTube video of a homemade 3-phase 2-pole motor spinning a coffee can!
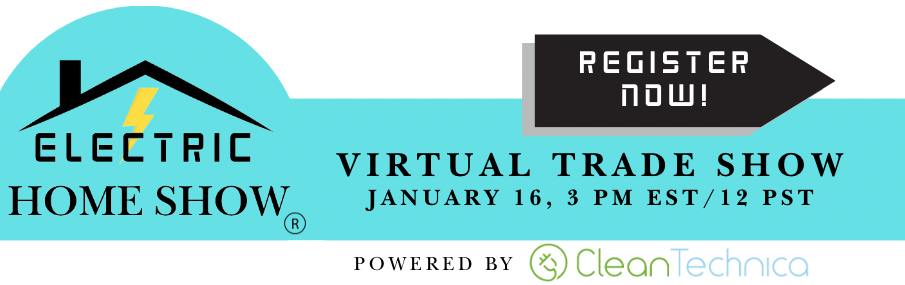
Chip in a few dollars a month to help support independent cleantech coverage that helps to accelerate the cleantech revolution!
Have a tip for CleanTechnica? Want to advertise? Want to suggest a guest for our CleanTech Talk podcast? Contact us here.
Sign up for our daily newsletter for 15 new cleantech stories a day. Or sign up for our weekly one if daily is too frequent.
CleanTechnica uses affiliate links. See our policy here.
CleanTechnica's Comment Policy